Imagine today is a significant day for your city. The government has announced a public-private partnership with several energy companies to install solar panels nearby. You feel optimistic about the future, hoping this initiative will reduce pollution and shift the electrical mix that powers your EV toward cleaner energy sources. However, your best friend, who works at the local research university’s research department, expresses concerns about the project.
He shares, “While I’m pleased we’ll be harnessing more electricity from cleaner sources like solar, I’m worried about the proposed installation site. It has a very low capacity factor—around 10%—which could lead to environmental issues affecting both crop production and natural habitats.”
This statement raises an important point. As informed citizens, we must consider not only the total installed power capacity of a project but also its capacity factors. The capacity factor measures a power plant’s effectiveness, indicating how fully it is utilized by comparing its average output to its maximum capacity.
To put it in perspective: for every 1 watt of fossil fuel-generated electricity, we need to install about 4 watts of solar power and 2 watts of wind power. As of 2022, our total installed solar energy capacity stood at approximately 1,053 GW, with China contributing the largest share at 363 GW. Yet, solar power generation worldwide for that year was only 1,323 TWh.
We can calculate the capacity factor using the following equation:
- Capacity Factor = Actual amount of power generation ÷ Maximum amount of power generation
Which would be equivalent to:
- Capacity Factor = 1,323 TWh ÷ (1,053 GW × 24 h × 365 days) = 14.34%
The capacity factor is defined as the ratio of electrical energy produced by a generating unit over a specific period to the electrical energy that could have been generated at continuous full power during the same timeframe. A capacity factor of 14.34% suggests that solar photovoltaic (PV) generation is not very efficient and raises important opportunity costs that must be considered when planning large-scale projects. In this article, I will discuss several factors to consider when new renewable investments, particularly in solar energy, are announced, along with potential solutions to mitigate associated negative effects.
Space Issues
Firstly, solar panels require significantly more space compared to traditional nuclear or geothermal plants. This land use can lead to soil erosion, disruption of natural ecosystems, and the destruction of habitats, which may reduce the availability of arable land for food production. In contrast, nuclear power plants in Europe and North America achieve average capacity factors of 83% and 85%, respectively, while geothermal plants reach 63% and 72%. These figures are markedly higher than those for wind (21% in the EU; 26% in North America) and solar energy (10% in the EU; 14% in North America). These disparities have crucial implications for infrastructure planning, particularly in meeting the growing demand for electricity and energy while maintaining living standards and preventing blackouts.
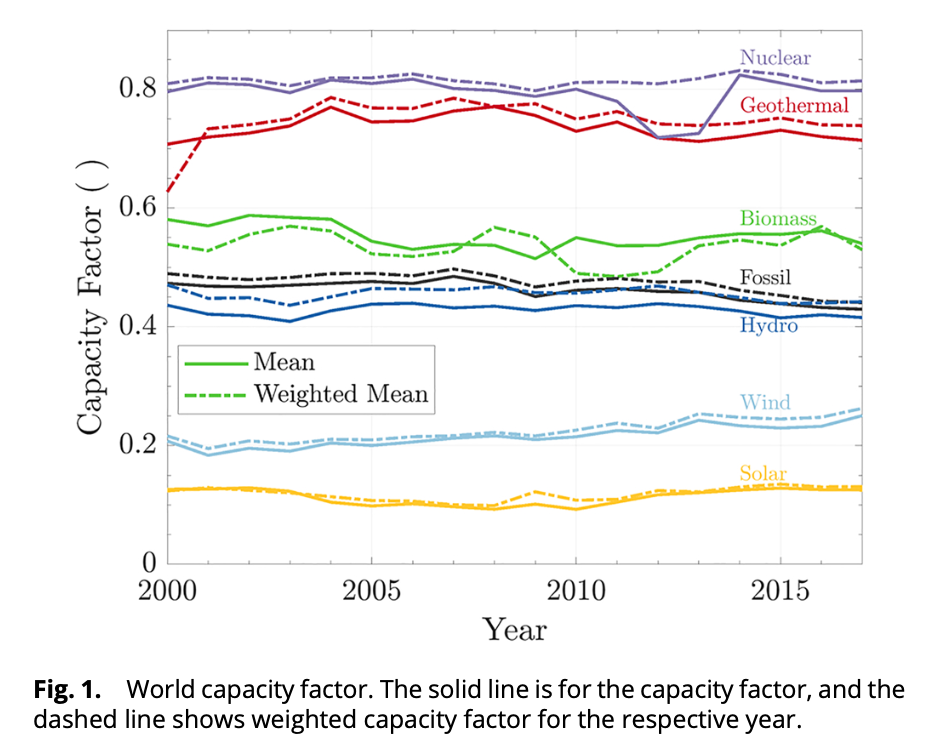
Research indicates that to replace the current fossil fuel capacity of 4 terawatts (TWe) solely with solar PV and wind at a 1:1 ratio, we would need to install 12 TWe from these renewable sources. This scenario assumes no growth in fossil fuel capacity, which does not account for the potential economic impacts of underutilization and foregone opportunity costs associated with a greater share of renewables in the energy mix. For instance, if regulations mandate a specific percentage of energy generation from renewables, this could lead to increased idle capacity in fossil fuel plants, resulting in economic losses and long-term operational challenges. These plants might become reliant on government subsidies, which means it is economically not efficient.
The installed capacity for renewable energy has significantly increased in recent years; however, the global share of electricity generated by renewables has been declining. This trend can largely be attributed to the low capacity factors associated with these technologies. In contrast, the share of global electricity generation from fossil fuels tends to align closely with their nominal capacity. Nuclear energy typically has a higher generation share compared to its global installed capacity, owing to its ability to operate continuously.
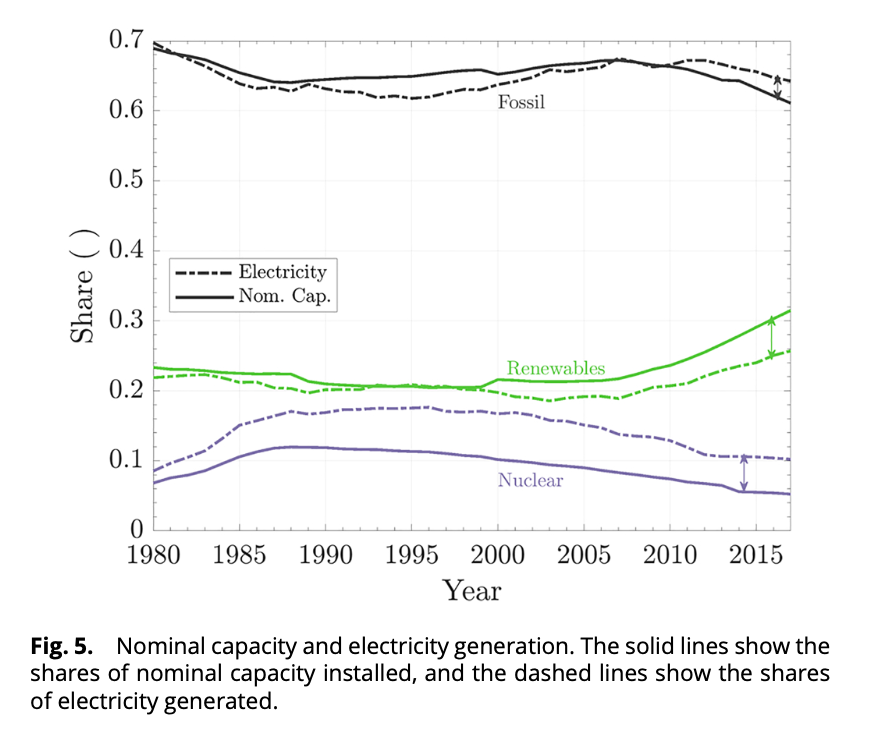
Sourcing Components
When considering the integration of renewables, particularly solar and wind, into the energy mix, the materials used for infrastructure are an important factor. For instance, if we envision a scenario where 12 TW of solar and wind power replaces fossil fuels, copper usage must be taken into account. Solar PV and onshore wind turbine systems can require up to 5 tons of copper per MW installed, while offshore wind systems may need as much as 10 tons. According to Bolson et al. (2022), this transition could necessitate around 75 million tonnes of copper. They also note that the current annual extraction rate is about 20 million tonnes, with reserves estimated at 870 million tonnes, suggesting a remaining lifespan of approximately 43.5 years.
Electrical Production Reliability
Bolson et al. (2022) also highlight another challenge: the unpredictable and intermittent nature of energy generation from these sources. Even the best statistical models struggle to accurately forecast wind speeds, solar irradiation, and cloud formations months in advance. This unpredictability complicates energy planning and forecasting, presenting significant challenges for industrial, commercial, and residential energy clients. Energy curtailment, where renewable energy production exceeds consumption, leads to wasted energy. Conversely, during peak energy demand, renewables may not generate enough power to serve as a reliable base load.
Recycling Mechanisms
Solar PV systems are becoming increasingly popular, not only among industrial consumers but also among residential users. In the U.S., home solar installations are estimated to have a combined capacity of 19 GW, with projections suggesting that this number could quadruple in the next decade. Government incentives, such as the Solar Tax Incentive Credit, can reduce tax liabilities by covering up to 26% of solar-related expenses. This policy, combined with rapid advancements in panel efficiency—improving by as much as 0.5% per year—and lower production costs, provides strong incentives for consumers to invest in solar power for their homes or to upgrade existing systems before their product lifespan expires.
While the progress in the energy transition is encouraging, there is a significant issue that often goes unaddressed: the fate of disposed solar panels. The International Renewable Energy Agency (IRENA) estimates that by the early 2030s, we could see substantial solar panel waste, potentially totaling up to 78 million tonnes by 2050. These figures, however, may underestimate the reality, as they assume that panels will last their entire recommended 30-year lifecycle.
Research from the Harvard Business Review suggests that if replacement costs are low and the additional kWh generated by newer models is compensated at a sufficiently high rate, rational consumers might be incentivized to replace their solar panels with newer, more efficient models before their expected lifespan ends.
HBR outlined three potential scenarios for solar waste generation:
Scenario 1: No failures occur, resulting in a significant increase in solar panel waste 30 years after widespread adoption (between 2010 and 2020), peaking around 2050.
Scenario 2: Regular product failures lead to some early replacements, forming the basis of IRENA’s statistical model.
Scenario 3: An early replacement model where consumers frequently switch to new solar panels, discarding older ones sooner than the indicated shelf-life due to decreasing prices and improving energy efficiency of solar products.
In the pessimistic scenario (Scenario 3), waste generation could exceed Scenario 2 by fourfold, translating to approximately 315,000 metric tonnes, based on an estimated weight-to-power ratio of 90 tonnes per MW.
The authors point out that solar recycling represents an untapped market opportunity, yet there is currently a lack of financial incentives to recycle solar panels. This can be attributed to several factors:
- The long lifespan of solar panels diminishes the motivation for innovative recycling solutions.
- The recent surge in solar adoption over the past decade means that large-scale recycling challenges have not yet emerged.
- The low cost of landfilling a panel (USD 1-2) compared to recycling (estimated at USD 20-30 based on research from First Solar) discourages recycling efforts.
- The specialized labor required for panel removal and the careful handling of hazardous materials, such as cadmium, further increases recycling costs.
If early replacement becomes common among residential and commercial customers, solar panel waste generated would be 2.56 times the number of solar panels currently sold in the U.S. This surge in waste would likely elevate the levelized cost of energy (LCOE), which is defined as the average cost per unit (kWh) generated by a system, calculated by the ratio of the total annualized cost of the system to the total electrical load served.
Moreover, this recycling challenge extends beyond solar energy; it also encompasses wind energy and electric vehicles (EVs). Reports indicate that approximately 720,000 tons of wind turbine blades are expected to end up in U.S. landfills over the next 20 years, and only 5% of EV batteries are currently being recycled.
Potential Solutions
Creating Multifunctional Spaces
In this article, I primarily addressed the challenges posed by low capacity factors associated with solar and wind energy. However, innovative solutions are being developed to mitigate the negative impacts of these technologies. One promising approach is the emergence of agrisolar businesses, which blend traditionally separate sectors like agriculture, feedstock production, and energy generation. A new breed of farmer or shepherd is now taking shape, as spaces once reserved exclusively for crops or grazing are being integrated with ecological solar panels.
These solutions require a multidisciplinary approach, incorporating expertise from topography, agricultural sciences, energy engineering, and meteorology. The goal is to create environments where solar photovoltaic (PV) panels can coexist with animals, trees, plants, and crops, aligning interests toward a shared objective: to generate sufficient energy for a growing population, enhance living standards, maintain food production, and protect local ecosystems.
New business and revenue models, referred to as “solar grazing” or “ecovoltaic” solutions, are emerging within this context. These models combine traditional agribusiness revenue streams from crop cultivation with cost-effective management of plants and grasses growing beneath solar panels, often involving shepherds and their sheep. This approach not only maintains the soil around solar installations but also optimizes the physical attributes of the panels to maximize crop growth while minimizing ecological disruption.
Adjustments can be made to variables such as panel height, array spacing for efficient water irrigation, and the installation of sun-tracking systems that tilt solar panels in accordance with the sun’s position. This enhances energy conversion efficiency while creating microclimates that adapt to sunlight patterns. For instance, closely spaced panel arrays can reduce water evaporation in arid ecosystems. In conclusion, integrating energy generation with crop production and farming presents a viable solution for optimizing land use, providing energy control for agricultural enterprises, and generating new income streams, all while offering a cost-effective strategy for field maintenance.
For a real-world example, see the collaboration of multiple stakeholders in the U.S.:
Creating a Circular Economy and Product Lifecycle Management
Let’s turn our attention to solar panels. As of 2021, only 10% of solar panels in the U.S. were recycled, a situation not mandated by federal regulations. While a handful of companies are beginning to explore the business potential of solar panel recycling, the task is fraught with challenges. Solar panels are complex, multi-layered technologies, with cells at their core. They consist of various materials, including silicon—a semiconductor that converts light to electricity—thin layers of metals (primarily silver), glass, and copper wiring. Protective barriers include transparent plastic known as EVA, an additional layer of glass, and PET plastic, all encased in an aluminum frame to shield against the elements.
The layered structure of solar panels complicates deconstruction when they reach the end of their lifecycle. As a result, reusing them is currently the most economically viable solution. According to MIT Technology Review, a new panel might cost around USD 55, while a used panel could be resold for approximately USD 22. If the components are sold separately, they could fetch a total of about USD 18. However, there is little incentive for customers to purchase second-hand panels, as they produce less electricity, and other associated costs (administrative, installation, etc.) comprise a larger portion of the total acquisition cost.
Some companies focus on extracting specific materials—such as glass, silicon, and other metals—transforming them into a mixture known as “glass cullet,” which can then be sold for various industrial applications. Nonetheless, economic viability remains a challenge, as this material typically sells for just USD 3.
According to Crownhart (2021), some startups, including ROSI Solar and Envie 2E Aquitaine, are working to establish more sustainable supply chains by recovering valuable solar components like silver and high-purity silicon—together accounting for 60% of a panel’s cost—before reselling them on the market. However, challenges persist, as profitability largely depends on the fluctuating market prices of these materials, which can be highly volatile.
Atasu et. al (2021) on “The Circular Business Model” describe several strategies that companies can undertake to make their business model more circular. These strategies include:
- Retaining business ownership
- Extending product lifetime
- Designing products with recycling in mind
Strategy choice, according to the authors, will depend on two factors:
- How much embedded value is left inside the product after “normal” usage by the immediate customer. These include asking questions such as: can the materials from my products be easily extracted and sold at a high price on the market? Is there a secondary market available for my product as it is? Can the product be reconditioned and reprocessed to serve new markets after its initial use?
- Can I retain product ownership during its lifetime? Is it easy to get my product back as a manufacturer?
A manufacturer is going to have an incentive to recycle and reuse its own produced product if it can extract substantial value from it. Products that have little value, like plastic bottles, need additional exogenous factors to incentivize recycling. For example, in Norway there is a large reverse supply chain network of vending machines that accept plastic bottles. Customers walk into those machines on the street and can make some little money based on the bottles that he gave in. These kinds of initiatives need large societal and government support on top of private investments in order to make them work. This is because one thousand plastic bottles is unlikely to make a significant impact on a recycling or manufacturing company but if the amount is scaled up to millions of plastic bottles and optimized pick up collection points are organized then this might become an economically profitable operation.
When considering wind turbines and solar panels, it’s essential to develop an effective strategy for these products. We recognize that recovering these items from a manufacturer’s perspective is challenging, as it involves high costs and complexities in extracting valuable materials such as minerals and metals. This process necessitates significant engineering capabilities and operational scale. Currently, there are no mature technologies capable of efficiently recycling all materials at a profit in other markets, to the best of my knowledge. Thus, I propose the following approach:
- In the short term, implement a product life extension and retention strategy within manufacturing while collaborating with regulators to foster a future recycling framework.
- In the long term, develop an international recycling and product reconditioning strategy aimed at achieving a nearly 90-100% circular business model within the next decade.
As countries globally continue to invest in wind and solar capacities, the demand for turbines and panels is expected to rise in the coming years. While product innovation enhances efficiency and durability, it also leads to rapid product turnover—akin to the release of new smartphones, where consumers quickly upgrade and discard older models. Manufacturers should adapt their business models to prioritize high-quality products that discourage frequent switching, thus ensuring that customers are less incentivized to transition to new panels upon the introduction of new brands. Additionally, providing excellent after-sales and maintenance services will create a win-win situation for both customers and the environment. Although initial costs for manufacturers may rise, fostering a loyal customer base can yield stable revenue streams, especially if periodic service and maintenance for turbines or panels are implemented.
Governments can play a pivotal role in promoting sustainable business models. Regulations could be established to require manufacturers to retain product ownership and offer lease contracts to energy companies or residential agents. Once a product’s lifespan is complete, manufacturers could buy back the item at salvage value from customers for recycling. This approach would necessitate significant R&D investments and collaboration among industry players to develop effective recycling programs.
Bibliography
Atasu, A., Duran, S., & Van Wassenhove, L. N. (2021, June 18). The dark side of solar power. Harvard Business Review. https://hbr.org/2021/06/the-dark-side-of-solar-power
Crownhart, C. (2021, August 19). What happens to solar panels when their useful life is over? MIT Technology Review. https://www.technologyreview.com/2021/08/19/1032215/solar-panels-recycling/
Atasu, A., Dumas, C., & Van Wassenhove, L. N. (2021). The circular business model. Harvard Business Review, July–August. https://hbr.org/2021/07/the-circular-business-model
Sturchio, M. (2023). Solar power occupies a lot of space—here’s how to make it more ecologically beneficial to the land it sits on. The Conversation. https://theconversation.com/solar-power-occupies-a-lot-of-space-heres-how-to-make-it-more-ecologically-beneficial-to-the-land-it-sits-on-216423
Stella, C. (2019, September 10). Unfurling the waste problem caused by wind energy. NPR. https://www.npr.org/2019/09/10/759376113/unfurling-the-waste-problem-caused-by-wind-energy
JMH Power. (n.d.). Solar energy vs. geothermal energy: The ultimate renewable energy battle. JMH Power. https://jmhpower.com/solar-energy-vs-geothermal-energy-the-ultimate-renewable-energy-battle/
Ritchie, H., & Roser, M. (n.d.). Solar energy consumption. Our World in Data. https://ourworldindata.org/grapher/solar-energy-consumption?tab=chart
Bolson, N., Prieto, P., & Patzek, T. (2022). Capacity factors for electrical power generation from renewable and nonrenewable sources. Proceedings of the National Academy of Sciences, 119(36). https://www.pnas.org/doi/epub/10.1073/pnas.2205429119